Jupiter (planet) - astronomy.
Publié le 11/05/2013
Extrait du document
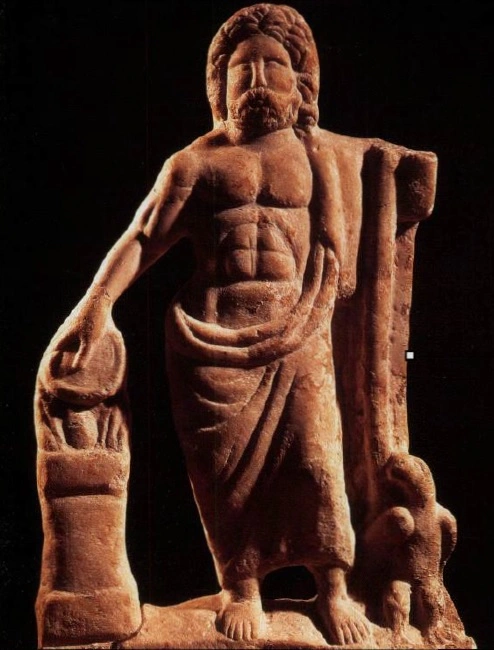
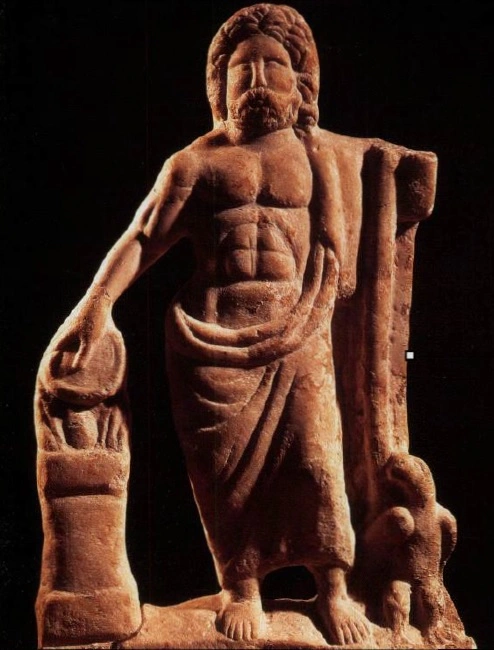
«
Beneath the supercritical fluid zone, the pressure reaches 3 million Earth atmospheres.
At this depth, the atoms collide so frequently and violently that the hydrogenatoms are ionized—that is, the negatively charged electrons are stripped away from the positively charged protons of the hydrogen nuclei.
This ionization results in asea of electrically charged particles that resembles a liquid metal and gives rise to Jupiter’s magnetic field.
This liquid metallic hydrogen zone is 30,000 to 40,000 km(19,000 to 25,000 mi) thick—about half the radius of the planet—and extends to the molten rock core at Jupiter's center.
The molten rock core occupies a sphere with aradius of about 10,000 km (about 6,000 mi)—about one-fourth of Jupiter's total radius—and has a mass perhaps 10 to 15 times the mass of Earth.
C Evolution of Jupiter
According to current theories, an enormous disk of dust and gas encircled the Sun as it formed about 4.6 billion years ago.
The material in this disk eventually formedthe planets, moons, and asteroids of the solar system.
Mineral particles and metal-rich grains in this disk combined with icy comet-like fragments to form seeds forlarger bodies.
The largest fragments swept up the most dust and surrounding gases and became the planets.
Planets such as Jupiter and Saturn that attained massesgreater than 14 times the mass of Earth had sufficient gravity to attract and hold hydrogen and helium atoms, which constituted most of the disk material.
Theseplanets became gas giants.
Planets with weaker gravity, such as Earth and Mars, could not hold hydrogen and helium and so remained smaller and mainly rocky.Eventually, nearly all of the matter of the disk was concentrated in a few bodies: the planets and their moons.
Jupiter was the largest of these bodies.
Despite the planet’s large size, Jupiter is far too small to become a star.
The pressure and temperature at Jupiter’s core are not high enough to cause sustained fusionof hydrogen—the process that makes a star shine.
Even though Jupiter contains more than twice as much mass as all the other planetary bodies in the solar systemcombined, it would need to have about 80 times its current mass for sustained fusion to occur.
Many puzzles remain about how Jupiter and similar giant planets form.
Scientists have been surprised by the orbits of some Jupiter-like planets discovered around otherstars.
In some cases, the giant planets orbit closer in around these stars than Mercury orbits our Sun.
Other Jupiter-like planets have extremely eccentric orbits, unlikeJupiter's nearly circular orbit.
These findings and other research suggest that Jupiter and the other giant planets in our solar system may not have formed in theirpresent orbital positions.
Jupiter may have formed closer to the Sun and moved outward, or Jupiter may have moved inward.
If Jupiter had a shifting orbit, itsmovements may have greatly affected the formation and orbits of other planets in the solar system.
How quickly Jupiter formed is another puzzle.
Planets are thought to form by accretion (clumping together) of smaller bodies while stars form by gravitational collapse atthe center of a gas cloud.
Accretion is thought to be a relatively slow process—too slow perhaps for Jupiter to have retained so much gas.
A faster way for a giantplanet to take shape might be the sudden collapse of a disk of dust and gas into a large body.
NASA’s planned Juno probe to Jupiter will study the size of Jupiter's rockycore, which may provide a clue to how Jupiter became a planet.
IV JUPITER’S ATMOSPHERE
As light travels outward from the Sun it spreads equally in all directions, decreasing in intensity.
Because Jupiter is five times more distant from the Sun than Earth is,the light that falls on Jupiter is 25 times less intense than the light that strikes Earth, and the intensity of solar energy reaching Jupiter is therefore only about 4 percentof that reaching Earth.
Studies of infrared radiation (energy radiated as heat) from Jupiter reveal that the planet gives off 1.67 times as much energy as it receivesfrom the Sun.
The source of the excess radiated energy is apparently stored heat that was created by the energy of impacts that occurred during Jupiter’s formationand the subsequent gravitational compression of the planet’s material.
The difference in temperature between the top of Jupiter’s atmosphere and its deepest layersdrives the circulation that transports heat from deep within the planet outward.
A Banded Appearance
From a distance Jupiter appears to have horizontal stripes, which result from winds that shear its cloud layers into sharply defined bands.
These bands circle the planet,with winds along the edges of adjacent bands blowing in opposite directions.
Earth’s trade winds form a similar pattern, but Jupiter’s winds are much stronger and morestable.
The strongest winds, at low latitudes near Jupiter’s equator, drive individual cloud systems 11° eastward every 24 hours.
At higher latitudes the cloudsalternately shift westward and eastward corresponding to the banded structure of the atmosphere, which is sculpted by these wind jets.
This cloud motion indicateswinds of 600 km/h (370 mph) at low latitudes with winds decreasing to tens of kilometers per hour at high latitudes.
Some of the cloud bands appear whitish, while others are orangey or brown.
Scientists believe that the colors result from the presence of trace gases in Jupiter’satmosphere.
In the upper reaches of the atmosphere, the temperature drops below the freezing point of ammonia, one of the trace gases.
In regions where warmergases are carried up from below, the fresh ammonia freezes to form highly reflective white ice crystals.
The ice crystals are swept horizontally by prevailing winds,causing the formation of bands that appear bright from reflected sunlight.
Ultraviolet radiation from the Sun interacts with molecules of other trace gases in the upperatmosphere and generates yellow-brown smog.
This smog settles down on the clouds causing those that are deeper in the atmosphere to appear darker brown.
Withinthe darker bands, the atmosphere tends to sink and the ammonia ice crystals melt, exposing more brown smog particles and causing further darkening.
B Storms
Major storms often appear suddenly on Jupiter.
Evidence suggests that, unlike storms on Earth, which are driven by solar heating of the atmosphere, Jupiter’s stormsare caused by bubbles of warmer gas rising through the atmosphere from deep within the planet.
These bubbles, carrying varying amounts of heat, create cloudsystems that are constrained on the north and south by bands of strong wind blowing in opposite directions.
Unable to move north or south, and with no solidlandmasses to create friction, the storms roll in the winds and feed off smaller storm systems for weeks or longer.
Jupiter’s most famous storm, the Great Red Spot, has persisted for centuries.
The Great Red Spot is so enormous that if three Earths were placed side by side in frontof it, they would scarcely span it.
The earliest report of a red spot was by Robert Hooke in 1664, although scientists are not sure if the current spot has existedcontinuously since that time.
The cause of the Great Red Spot is not yet known, but its motion is such that it must sustain itself on energy gained from the upperatmosphere, perhaps by absorbing the energy of smaller atmospheric disturbances.
It cannot be linked to a heat source deep in the atmosphere, because it movesslowly westward at an irregular rate.
The red color of the spot appears to be caused by impurities such as sulfur or phosphorus compounds that absorb ultraviolet,violet, and blue light.
In 1938, three smaller, separate storms formed in a belt near 30° south latitude.
Because of their color and shape, these storms were called white ovals.
In 1998astronomers observed that two of these white ovals had merged to form a slightly larger storm system, visible as a single white oval.
In 2000 the remaining two stormsystems combined into a single storm with a diameter half that of the Great Red Spot.
The storm was rotating in a counterclockwise direction as seen from above.Weather systems on Earth that behave in this manner have air masses rising near their centers.
In late 2005, the storm turned brown and in early 2006 it turned red.
Scientists dubbed it the Little Red Spot, although the official name is Oval BA.
It was the first timescientists were able to witness the birth of a red spot on Jupiter.
The color change probably resulted from chemicals being pulled into the upper atmosphere by thestorm and exposed to ultraviolet radiation from the Sun.
By late 2006 the winds in the Little Red Spot were blowing at about 650 km/h (400 mph), similar to wind.
»
↓↓↓ APERÇU DU DOCUMENT ↓↓↓
Liens utiles
- Earth (planet) - astronomy.
- Mars (planet) - astronomy.
- Mercury (planet) - astronomy.
- Neptune (planet) - astronomy.
- Uranus (planet) - astronomy.